Lipid Nanoparticles
Lipid nanoparticles (LNPs) are non-viral drug delivery systems. LNPs are designed to encapsulate and transport bioactive molecules such as RNA-based drugs (e.g., mRNA and siRNA) and gene editing tools (e.g., CRISPR-Cas9) into target cells in vitro or in vivo. LNPs offer several advantages as a cellular delivery system, including biocompatibility, stability, consistency, scalability, and versatility in terms of payload options. They have demonstrated potential in various diseases and mRNA vaccine development.
Cat. No. | 产品名称/活性 |
---|---|
7945 | Cholesterol |
Component of lipid nanoparticles (LNPs); modulator of lipid bilayer fluidity | |
7176 | DC-Cholesterol hydrochloride |
Cationic cholesterol derivative | |
7946 | DLin-MC3-DMA |
Component of lipid nanoparticles (LNPs); ionizable cationic lipid | |
7944 | DMG-PEG 2000 |
PEGylated myristoyl diglyceride; commonly used in the formation of lipid nanoparticles (LNPs) | |
7175 | DOPE |
Liposome/lipoplex transfection enhancer | |
7179 | DOTAP |
Cationic agent for liposome and LNP formation | |
7943 | DSPC |
Neutral phospholipid; component of lipid nanoparticles (LNPs) | |
8837 | DSPE-PEG 2000 Azide 最新 |
PEGylated DSPE with azide reactive group | |
8838 | DSPE-PEG 2000 Biotin 最新 |
Biotinylated DSPE PEG lipid | |
7947 | DSPE-PEG 2000 Maleimide |
PEGylated DSPE with a maleimide reactive group | |
8889 | MC3 LNP Luciferase 最新 |
Dlin-MC3 containing LNP for in vitro and in vivo delivery of luciferase mRNA |
What are Lipid Nanoparticles?
Lipid nanoparticles (LNPs) are lipid based delivery systems commonly used for delivering bioactive nucleic acid payloads into living cells. LNPs are nanometer-sized particles (< 100 nm) comprised of a lipid monolayer shell surrounding an internal core packed with cargo molecules encapsulated in reverse micelles.
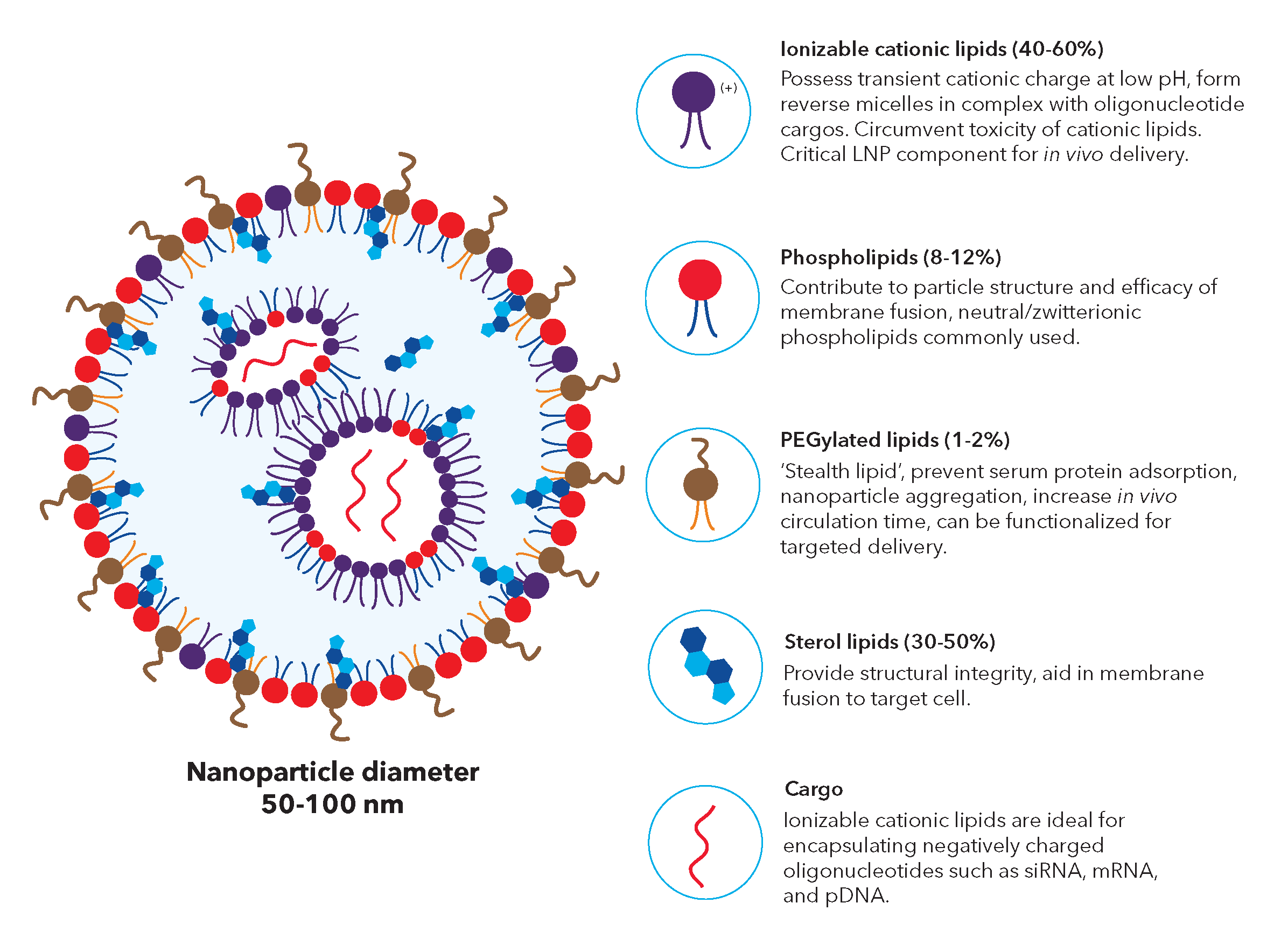
Figure 1: Structure and component of lipid nanoparticle carriers. Nucleic acid cargos are stabilized by the ionizable lipids and contained in reverse lipid micelles inside the nanoparticle. The outer membrane with PEGylated lipids provides protection for the core.
There are four essential components of LNP formulations:
- Ionizable lipids typically contain a tertiary amine that is deprotonated at physiological pH and becomes cationic in pH conditions below the acid-dissociation constant (pKa) of the lipid, e.g., in mature endosomes (pH between 5.5 and 4.5). They serve two key functions including facilitating nucleic acid encapsulation in LNPs and mediating endosomal membrane disruption (endosomal escape) to enable nucleic acid release to the cytosol. Ionizable lipids also play an important role in endosomal uptake, either directly through interaction between the positive charge on some ionizable lipids and the negatively charged cell membranes or via their binding to plasma proteins that support cellular uptake.
- PEG-lipids usually contain two fatty acid chains and a linear polyethylene glycol (PEG) molecule covalently attached to the head group of the lipid. During LNP formation, PEG-lipids promote LNP self-assembly via the hydrophilic steric barrier and are essential for preventing aggregation. The morphology of the LNP is affected by the molar ratio (mole fraction) of the PEG-lipid in the LNP synthesis mixture, and both the structure and length of the fatty acid and PEG chains. In turn, these factors affect the characteristics of the LNP such as nucleic acid loading efficiency, circulation time, immunogenicity, and transfection efficiency. A reactive handle such as maleimide can be incorporated onto the LNP surface by using functionalized PEG-lipids, on which the reactive group is attached to the free end of the PEG component.
- Phospholipids contain a glycerol backbone with a hydrophilic head group and two hydrophobic fatty acyl chains. Saturated phosphatidylcholine lipid, DSPC (distearoylphosphatidylcholine), is the most prevalent neutral phospholipid in LNP formulations. It has a high phase transition temperature (Tm, the temperature required to induce a change in the lipid physical state from the ordered gel phase to the disordered liquid crystalline phase) with proven stability in commercial liposomes. Phospholipids have several different functions in LNPs including assisting cellular delivery and encapsulation.
- Sterol lipids, such as cholesterol, play roles in LNP formation depending on context. In formulations containing phospholipids with low gel-liquid crystalline Tm, sterol lipids decrease membrane fluidity and increased bilayer thickness, thereby helping the formation of the liquid-ordered phase and increasing membrane stability. In high Tm lipids, sterol lipids reduce the thickness of the bilayer and pull the lipids towards a liquid-ordered phase, thereby increasing membrane fluidity. Sterol lipids are required to facilitate stable nucleic acid encapsulation.
Table 1. List of LNP components available from Tocris Bioscience
LNP component | Product | Cat. No. |
---|---|---|
Ionizable lipid* | DLin-MC3-DMA | 7946 |
Phospholipid* | DSPC | 7943 |
PEG lipid* | DMG-PEG 2000 | 7944 |
Sterol lipid* | Cholesterol | 7945 |
Cationic lipid | DC-Cholesterol hydrochloride | 7176 |
Functionalized lipid | DSPE-PEG 2000 Azide | 8837 |
DSPE-PEG 2000 Biotin | 8838 | |
DSPE-PEG 2000 Maleimide | 7947 | |
Fully formulated LNPs | MC3 LNP Luciferase | 8889 |
*Essential components for LNP synthesis. For more information on how to prepare MC3 lipid nanoparticles for RNA delivery, please see our protocol.
Compared to other nanocarriers, such as liposomes, LNPs offer several advantages. They are more stable, less fusogenic, and can be manufactured with low batch-to-batch variation. LNPs are also less cytotoxic, less immunogenic, and have a high encapsulation efficiency of nucleic acids with increased transfection efficiency.
Applications of LNPs in Genetic Medicine
Genetic medicine has a wide range of applications including vaccines, cancer immunotherapy development, and treatment of rare genetic and undruggable diseases. There is an increasing number of nucleic acid-based drugs, such as short messenger RNA (mRNA), small interfering RNA (siRNA), and gene editing constructs, including some that have been approved by the FDA. However, there are major hurdles for developing nucleic acid-based drugs: they are large, polar, and metabolically unstable. The rate of cellular uptake of unmodified oligonucleotides is extremely low. Moreover, RNA usually has a short intracellular half-life and is readily broken down by exonucleases and endonucleases commonly found in biological fluids. Reliable delivery systems are therefore essential for any genetic medicine to reach its target site.

Figure 2: In vivo delivery of oligonucleotides to hepatocytes using LNPs. Endogenous ApoE in the blood compartment is recruited to the LNP surface after IV administration. The ApoE-LNPs are recognized by ApoE-binding receptors on hepatocytes resulting in uptake of the LNPs into the cell by endocytosis. The ionizable lipids are protonated at low pH in the endosome, which leads to the destabilization of the endosomal membrane and the release of the payload into the cytoplasm.
LNP systems are commonly used in clinically advanced non-viral gene delivery systems. They offer several advantages over viral delivery systems: they are relatively easy to synthesize, inexpensive to manufacture, and straightforward to scale with consistent efficiency. Furthermore, the use of LNPs for gene delivery has improved the safety profile of genetic therapies in vivo.
Patisiran, the first small siRNA-based drug to receive FDA approval, is an LNP formulation of siRNA for the treatment of polyneuropathies resulting from the hereditary disease transthyretin-mediated amyloidosis (hATTR); it inhibits the synthesis of the transthyretin (TTR) protein in the liver. LNPs enable in vivo delivery of encapsulated siRNA to the cytoplasm of hepatocytes following intravenous (IV) administration (Figure 2). Once inside the target cells, the siRNA can target the conserved region of TTR mRNA and reduce levels of both wild-type and pathogenic TTR protein. The LNP vehicles consist of DLin-MC3-DMA (Cat. No. 7946), DSPC (Cat. No. 7943), DMG-PEG 2000 (Cat. No. 7944), and Cholesterol (Cat. No. 7945). In the case of Patisiran, the ⁓100 nm particle size favors extended circulation in the bloodstream, and the narrow sinusoidal fenestration pores in the liver have an average diameter of 100 nm, so LNPs with a diameter of 100 nm or less are ideal for this application. LNP size can be adjusted by changing the proportion of surface PEGylated lipid to core lipid (the higher % of PEG-lipid, the smaller the LNPs). Using 1.5 % DMG-PEG 2000 can produce LNPs with diameters of 100 nm or less. PEG coating on the LNP surface has the disadvantage of inhibiting the interaction of the LNPs with serum proteins and target cells; DMG-PEG 2000 has relatively short C14 acyl chains to overcome this problem. The chemical and physical properties of PEGylated lipid enhance the liver-targeting characteristics of LNPs: It has been demonstrated that in the presence of a lipid sink, such as lipoprotein particles in blood plasma, the PEG-lipids can exchange out of the LNPs and the outer membrane can become unshielded, which enables the recruitment of endogenous apolipoprotein E (ApoE) from the circulation to the LNP surface. ApoE acts as a highly effective targeting ligand by binding to lipoprotein receptors on hepatocytes and triggering intracellular uptake of the LNPs by endocytosis. DLin-MC3-DMA is another key component for locking and unlocking the payload in the right place. During the mixing/synthesis step, it provides positive charges to stabilize the negatively charged siRNA in the core. At physiological pH, DLin-MC3-DMA becomes nearly neutral and the resulting LNPs display low surface charge and are relatively non-toxic and non-immunogenic. After the LNPs are taken up by endocytosis, more of the ionizable lipids in the LNPs become positively charged as the pH of the endosome starts to drop. The protonated DLin-MC3-DMA interacts with negatively charged endogenous lipids to disrupt the bilayer membrane and results in cytoplasmic delivery of siRNA. This LNP system allows for a readily scalable manufacturing process, which has also allowed it to be used in the development of two COVID-19 mRNA vaccines (BNT162b2 and mRNA-1273).
Future Direction of LNP Research: Tissue-Specific Delivery
IV-administered LNPs are typically internalized by hepatocytes and accumulate in the liver, which greatly limits their applications. Delivery systems allowing cellular targeting are a research focus for the field of nanomedicine. For example, in cancer immunotherapy, chimeric antigen receptor (CAR) T cell therapy has shown promise in metastatic and blood cancer treatment. These T-cells are genetically modified to express transgenic T cell receptors (TCRs) to specifically target and eliminate cancerous cells. However, CAR-T cells are typically generated ex vivo, which can be costly. In addition, T cells are notoriously resistant to transfection by exogenous mRNA. Tombácz et al. demonstrated that CD4-targeted LNPs can be synthesized by simply adding two extra steps to the common procedure: after mRNA payloads are encapsulated in LNPs, DSPE-PEG 2000 Maleimide (Cat. No. 7947) is incorporated onto the outer membrane by a post-insertion technique. The antibodies (Abs) specific for CD4 can then be conjugated to LNPs via SATA (N-succinimidyl S-acetylthioacetate)-maleimide conjugation chemistry. After systemic injection of the Abs-LNPs-mRNA construct in mice, reporter gene expression is shown in about 60% and 40% of CD4+ T cells in the spleen and lymph nodes, respectively. This LNP-based T cell-targeted mRNA delivery system provides a robust and rapid method for in vivo T cell manipulation.
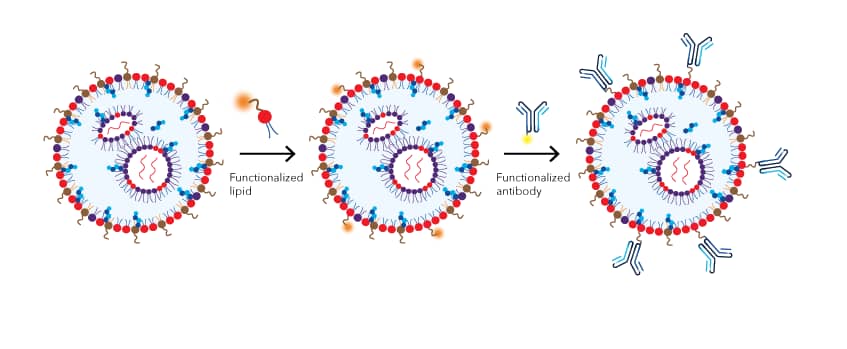
Figure 3: Incorporating a targeting moiety onto the LNP surface. A two step reaction is used to preserve the functions of the targeting moiety. In the first step, functionalized lipids form micelles that preferentially insert into the outer membrane of the LNP and introduce the reactive chemical groups (e.g. maleimide) to LNP surface. In the second step, the targeting moiety, such as an antibody, is conjugated to the surface of the LNP via the reactive groups.
With a reactive chemistry handle, such as DSPE-PEG 2000 Maleimide, incorporated into the LNPs surface, other targeting moieties, including antibodies, peptides, integrin ligands, glucose, transferrin, and folic acid can be conjugated to the LNPs for a wide range of possible applications.
Dammes et al. incorporated a conformation-sensitive targeting moiety to the surface of LNPs. DSPE-PEG 2000 Maleimide was added to the initial lipid mixture in the synthesis/packing step, and a microfluidic device was used to ensure the consistency of LNP production. The recombinant fusion portion with integrin binding domains D1 and D2 were then conjugated to the LNPs via maleimide/thiol chemistry. The resulting MAdCAM-D1D2-LNP- siIFNγ construct was designed to target the high-affinity conformation of α4β7 integrin, which is a hallmark of inflammatory, gut-homing leukocytes. The efficacy of interferon γ silencing specifically in the gut was demonstrated, which then led to an improved therapeutic outcome in the piroxicam-accelerated colitis (PAC) murine model.